Microfluidics for Cytology Heading link
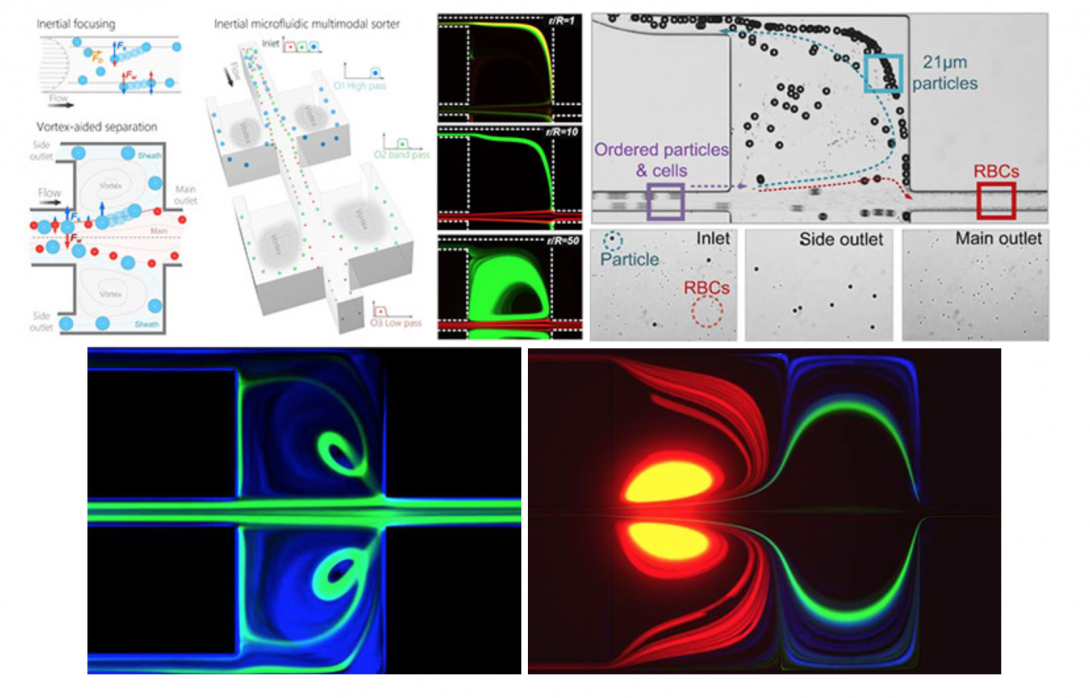
Inertial microfluidics shows tremendous potential for high-throughput cell separations in biomedicine. The approach relies on hydrodynamic forces to manipulate cells in microfluidic channels for size-based focusing and ordering. The interaction of these forces on cells in flowing fluid leads to lateral migration across streamlines into specific cross-sectional positions depending on their size, shape, and deformability. Recent studies demonstrated these effects in microchannels of various geometries, including straight, spiral and serpentine channels. Most inertial microfluidic devices are only capable of bimodal separation with a single cutoff diameter and a well-defined size difference. These limitations inhibit efficient separation of complex real-world samples, which often include heterogeneous mixtures of multiple microparticle components. Further, precise adjustment of the separation cutoff in most devices is challenging, and often requires re-design and re-fabrication of device geometry. To address these shortcomings, we are developing microfluidic devices for continuous multi-modal sorting of cells with high resolution and high tunability of the separation cutoff diameters. Our ultimate goal is to sort complex cellular samples in devices that allow easy integration with downstream detection. Ultimately, the unique separation functionalities of inertial microfluidics broaden applications of microfluidics in sorting of complex cell mixtures, from liquid biopsy to systems biology.
Microfluidics for Hematology Heading link
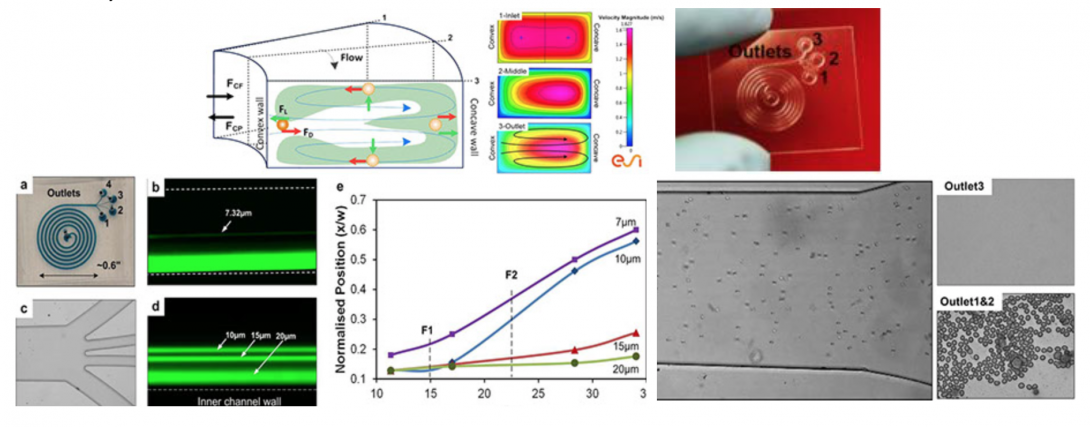
Blood fractionation is critical to sample preparation for both clinical diagnosis and research. We have developed the spiral inertial microfluidic devices that can achieve label-free, continuous separation of cell mixtures with high throughput and efficiency. The devices utilize hydrodynamic forces acting on cells within laminar flow, coupled with rotational Dean drag due to curvilinear microchannel geometry. Our current design uses an optimized Archimedean spiral devices to achieve cell separation in less than 8 cm of downstream focusing length. These devices are small in size (<1 in2), exhibit high separation efficiency (95%), and high throughput (1–2ml/min, 0.1% 45% hematocrit) with rates up to 106 cells per minute. These device concepts offer a path towards possible development of a lab-on-chip for point-of-care blood analysis with high efficiency, low cost, and reduced analysis time.
Microfluidics for Cytometry Heading link
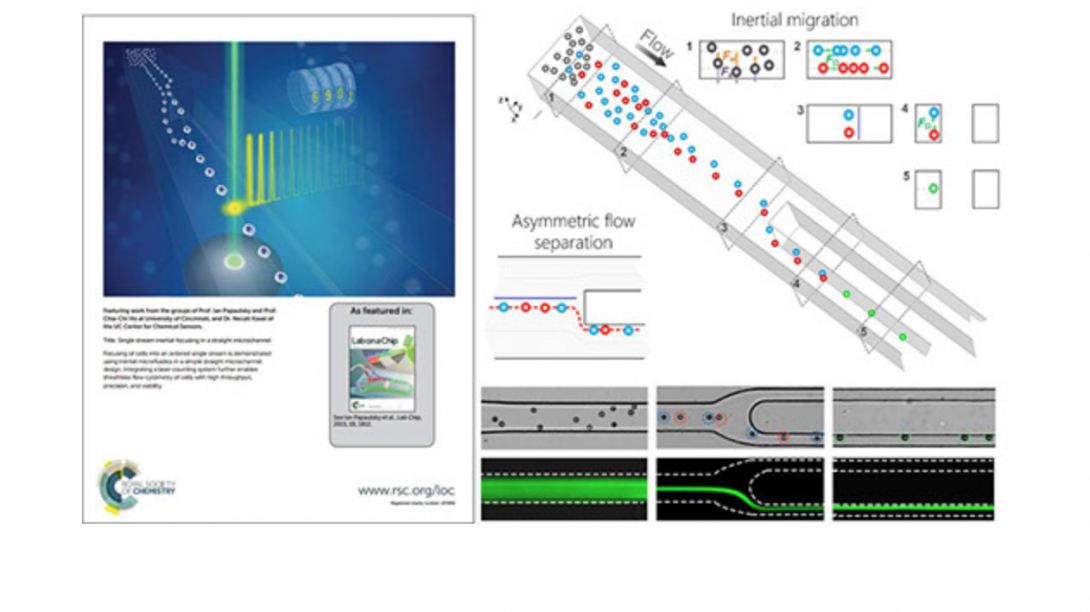
Microfluidic techniques use either external forces or sheath flow to focus particulate samples, and face the challenges of complex instrumentation design and limited throughput. We are developing an inertial microfluidic focuser that uses inertial lift force as the only driving force to focus microparticles into a single position. We demonstrate single-position focusing of different sized microbeads and cells with 95~100% efficiency, without the need for secondary flow, sheath flow or external forces. We further integrate this device with a laser counting system to form a sheathless flow cytometer, and demonstrated counting of microbeads with 2200 beads/s throughput and 7% coefficient of variation. Cells can be completely recovered and remain viable after passing our integrated cytometry system. Our approach offers a number of benefits, including simplicity in fundamental principle and geometry, convenience in design, modification and integration, flexibility in focusing of different samples, high compatibility with real-world cellular samples as well as high-precision and high-throughput single-position focusing.
Point-of-care Electrochemical Sensors Heading link
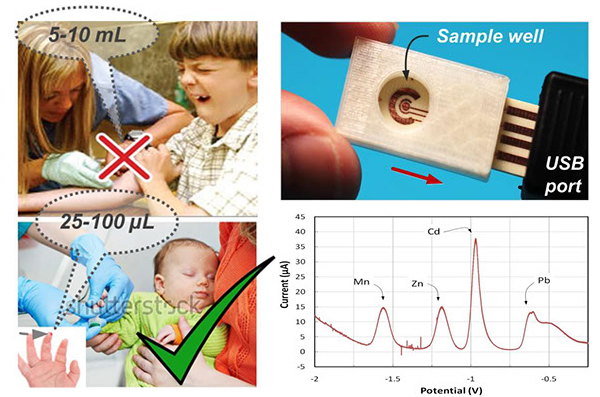
Metals are ubiquitous in the environment and have long been recognized to pose significant threats to human health. There is a critical need for rapid and accurate on-site measurement of metals exposure in susceptible pediatric populations. Biomarkers of pediatric, low-level exposure to lead (Pb), manganese (Mn), and cadmium (Cd) are necessary to quantitatively assess and identify adverse consequences of these exposures. Current approaches suffer from high costs including extensive labor, equipment and time-consuming laboratory procedures, and often demonstrate long turnaround times. To addresses this need we are developing sensor technology by integrating electrochemistry with microfluidics. Electrochemistry is a well-established technique that can significantly benefit from miniaturization. Microfluidics can be used to integrate sample preparation, such as blood cell extraction or sorting, directly on-chip. The ultimate vision is an environmentally-friendly sensor capable of point-of-care multiplex metal analysis for exposure assessment of susceptible populations in national and international settings.
Point-of-care Biosensors Heading link
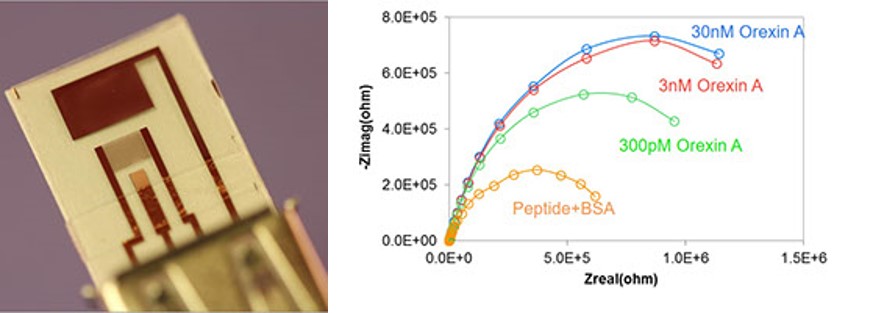
Electrochemical impedance spectroscopy (EIS) can offer a relatively simple but highly sensitive electrochemical biosensing approach to determination of biomolecules. EIS sensors are often based on a two-electrode electrochemical cell and consist of interdigitated electrode array (IDA) electrodes functionalized with a bio-recognition element (e.g., antibody, peptide, aptamer) which provides high level of selectivity. Targets are captured by the bio-recognition elements, which induces change in sensor impedance. The Faradaic measurement can then be used to relate biosensor impedance to the target concentration. Our recent work shows that this approach can be used for sensitive detection of neurotransmitter Orexin A, in the pM range.